Authors: M. Dietz1, A. Di Chicco1, R. Nolte1, E. Pirovano1 and the n_TOF collaboration2
1) Physikalisch-Technische Bundesanstalt (PTB), Germany
2) European Organization for Nuclear Research (CERN), Switzerland
Introduction
The CERN spallation source n_TOF offers a broad neutron spectrum from thermal to GeV neutrons and considering the scarcity of high neutron energy facility (> 20 MeV), n_TOF is the unique neutron source in Europe for neutron-induced experimental studies in the energy range of hundreds of MeV. For identification of reaction products like light charged particles in (n, lcp) experiments, multistage particle-telescopes are exploited consisting of semiconductors and plastic scintillation detectors of different thicknesses. Employing semiconductor detectors at pulsed ultra-strong neutron sources, such as n_TOF, presents many challenges.
In this work, the focus was on the electromagnetic noise associated with the ‘gamma flash’, i.e., the flash of high-energy photons and relativistic particles that accompanies every neutron pulse. The effects of gamma-flash-induced noise on the read-out electronics can range from a deterioration of the energy resolution up to causing large oscillations in the read-out signal, with amplitudes comparable or higher than the pulse height of particle-induced events.
Development stages
In 2022, two detector tests were carried out at n_TOF to investigate the best configuration for the read-out electronics of silicon semiconductor detectors, which are intended to be used to detect charged particles produced by neutron-induced reactions [1]. In previous experiments, the gamma flash was found to partially exhaust the dynamic range of the charge-sensitive preamplifiers connected to the detectors, consequently leading to the loss of valid events due to early saturation.
In the first detector test in May 2022, the intensity of the gamma flash did not pose a problem per se, however it did still significantly disturb the data acquisition, as it was found to be associated with electromagnetic noise that produced oscillations in the read-out signals with amplitudes comparable to the pulse height of particle-induced events. Figure 1 (left) gives an example of this radiofrequency (RF) noise dominantly present in the first ten microseconds after the gamma flash. The issue was solved in the second test in November 2022 as illustrated in Fig. 1 (right), during which the preamplifiers and shaping amplifiers were mounted in the same vacuum chamber as the silicon diodes, to minimize the distance from the detectors and to use the chamber itself as an electromagnetic shield.
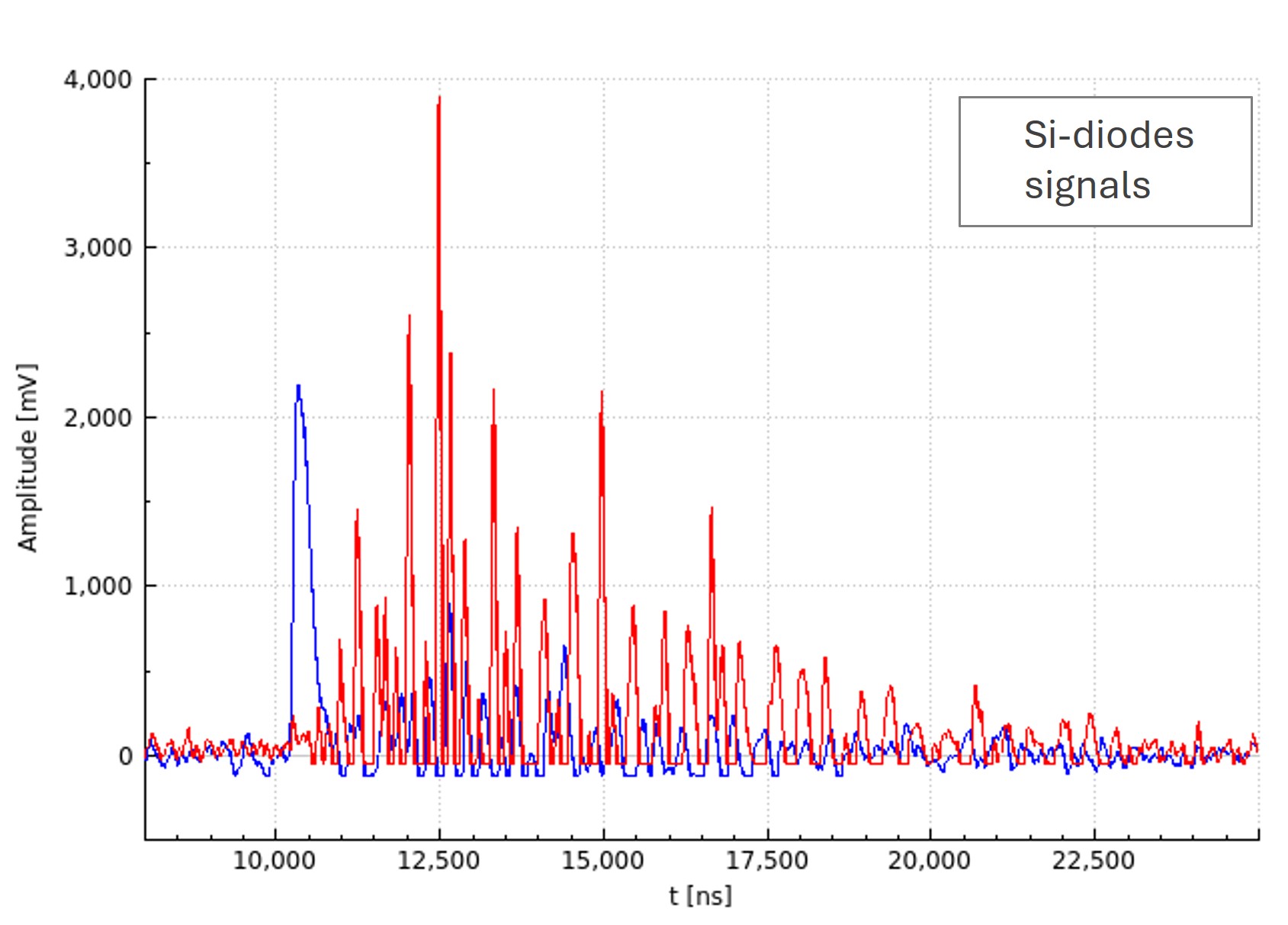
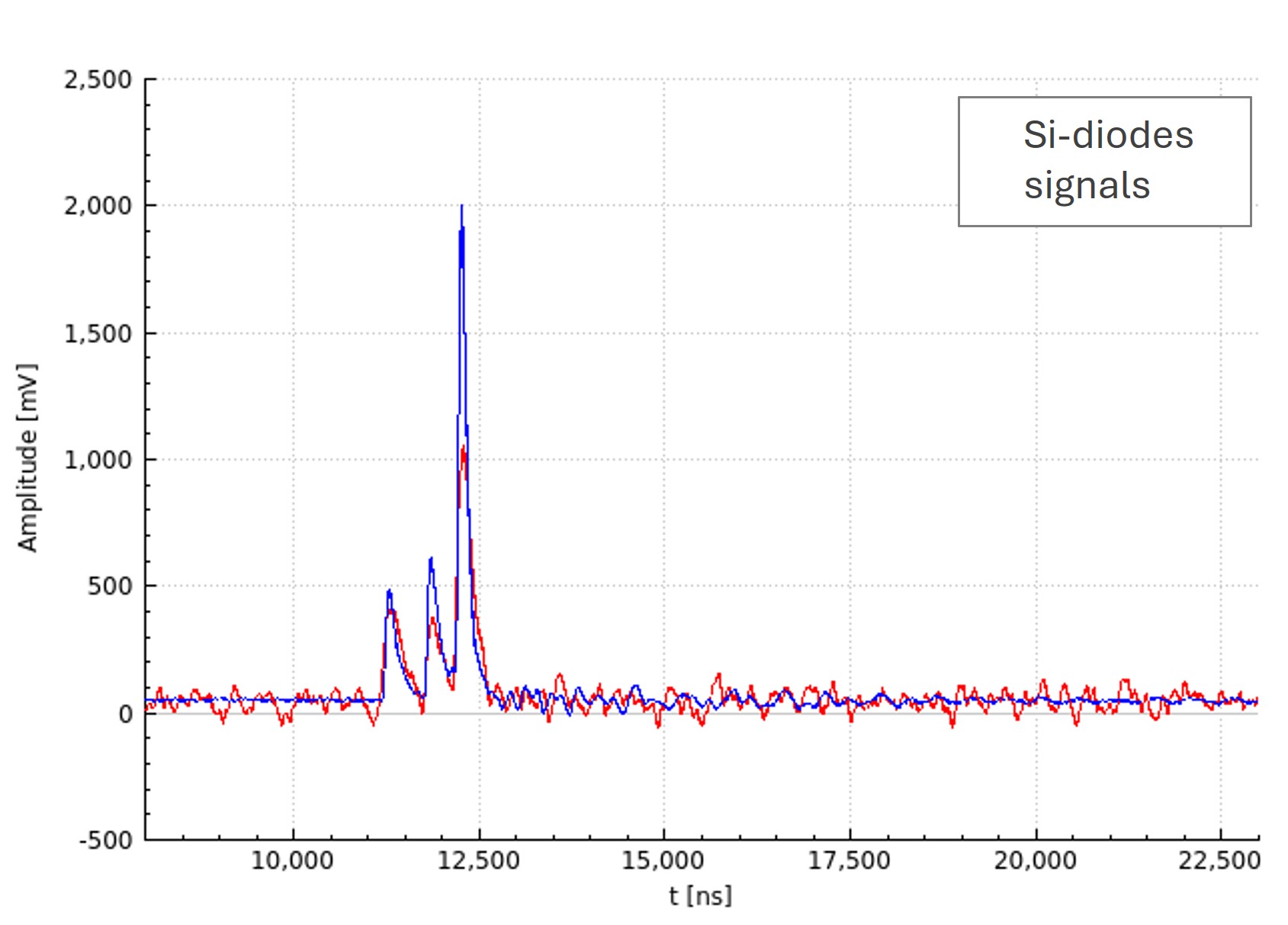
Figure 1: Examples of wave form signals from silicon diodes with RF-noise dominant signals in first test (left) and clean particle-induced signal with obvious events of interest (right) from the second test, where (pre)amplifiers were mounted within RF-tight chamber.
Therefore, the electromagnetic noise was further investigated among others by CERN impedance group, which found electromagnetic interferences peaking in the frequency range from 2 MHz to 7 MHz. To shield the read-out early in the signal chain, the Kapton windows of the vacuum chamber were altered to include an additional aluminium foil of 100 µm thickness. This was a compromise between the noise reduction on the semiconductor detectors and the introduction of an additional dead layer between the second silicon diode and the third stage of the telescopes, which is usually a plastic scintillator outside of the chamber.
The design of the supports of the silicon diodes was constantly improved as shown in Fig. 2, starting from unshielded cables to shielded cables and the replacement of the pin connectors with SMA connectors for a more stable configuration. The PCB board was extended to house the preamplifier and shaping amplifier close to the diode with short direct connections. Amplifier modules of Cividec (Cx-L series) and of Cremat Inc, charge-sensitive preamplifier module CR-11x and shaping amplifier CR-200-x with high gain and short integration time, were found to be best suited [2]. To increase the resolution of the thin silicon diodes, a segmentation was tested to reduce the capacity of the diode and therefore also its noise level in the read-out.
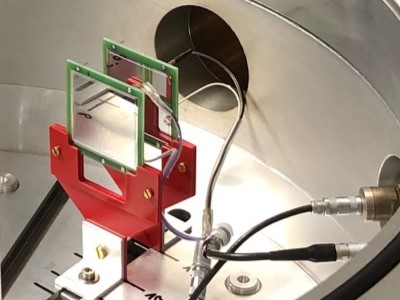
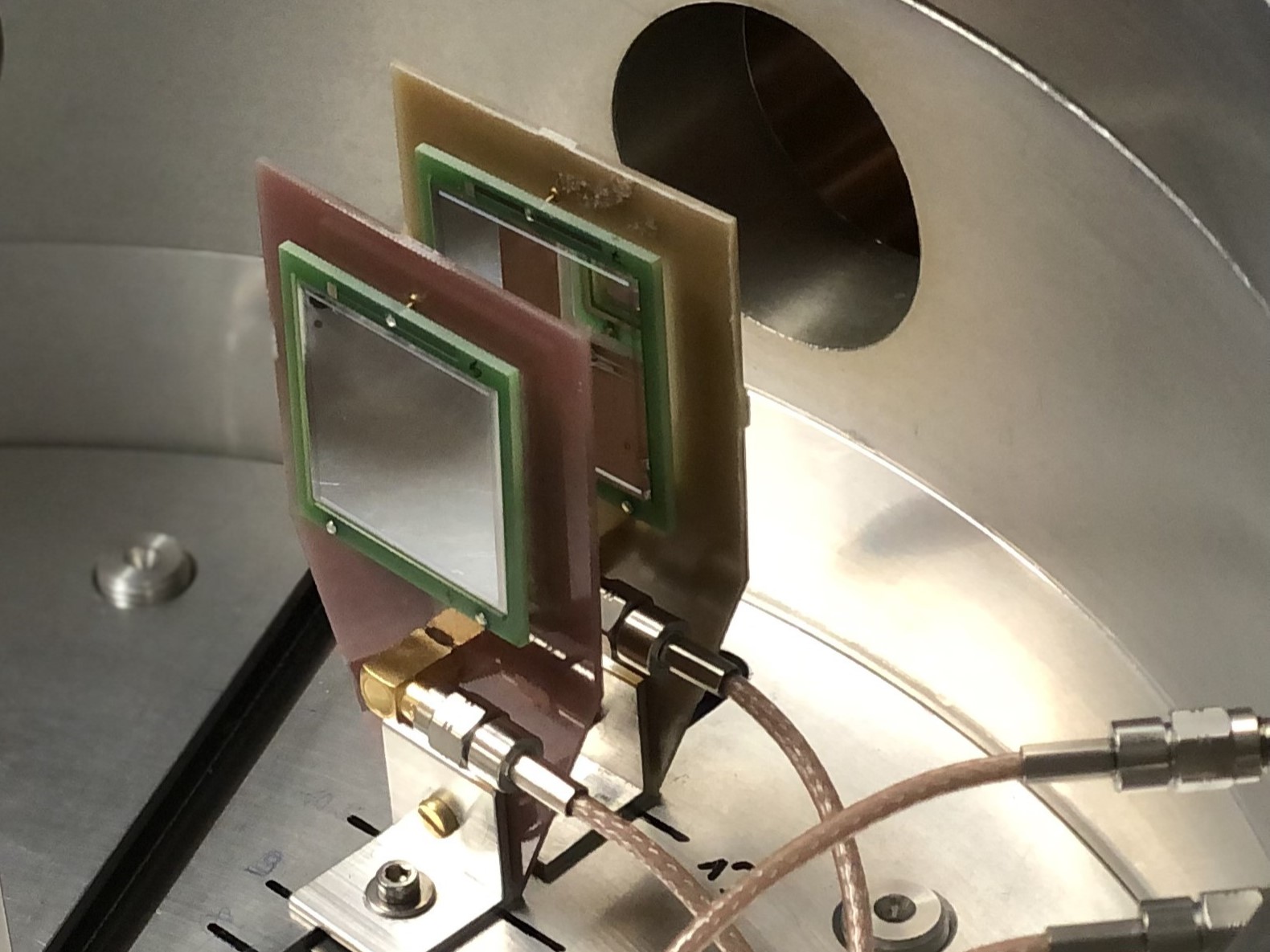
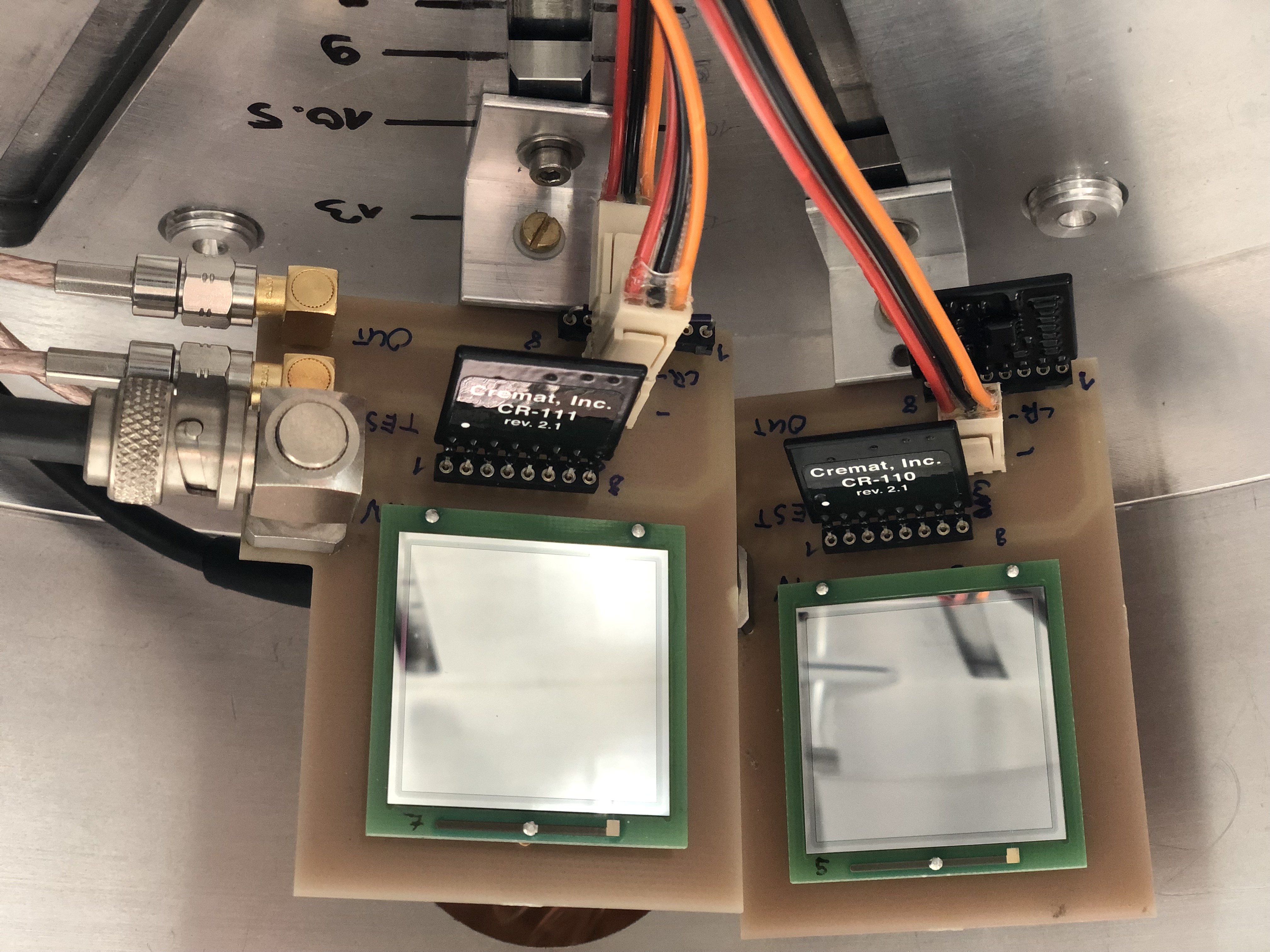
Figure 2: Pictures of the diode holders and connectors in the first detector test in May 2022 (left) and in November 2022 (middle and right). Pin connectors were exchanged with SMA connectors, shielded cables were used and the Cremat (pre)amplifier modules were installed on the PCB board inside the chamber.
Finally, all these findings about the shielding and the read-out of the semiconductor were accumulated in the design of a new chamber for the kind of neutron-induced reaction studies at high neutron energies.
Experimental setup with new chamber
Between September and October 2024, a proof-of-principle measurement [3] of double-differential cross sections (DDX) data for the emission of light charged particles on carbon at continuous neutron energies from 20 MeV to 200 MeV was carried out at the neutron time-of-flight facility n_TOF at CERN. Figure 3 (left) shows the experimental setup at n_TOF EAR1 with the scattering chamber and five surrounding plastic and inorganic scintillation detectors to measure the light charged particles (p, d, t, 3He, α) from the neutron-induced interaction on the graphite sample. The new experimental chamber housed nine silicon diodes (from Micron and Canberra) with thicknesses between 50 µm and 1000 µm plus one segmented silicon diode with 60 µm. The read-out was mostly with Cremat modules and partially with additional Ortec 579 Fast-Filter Amplifier for flexible amplification settings. The chamber was decoupled from the aluminium stand and the electronic grounding was only achieved via the signal / power cables and their SMA / BNC connectors.
Figure 3 (right) shows e.g., a particle detector telescope on the left with a segmented diode coupled to four read-out-channels with CR-110 on a customised PCB board and a 500 µm diode behind it as ΔE1 and ΔE2 detector. As a third E-detector a EJ-204 plastic scintillator of certain length is placed outside the chamber behind the Kapton window, but the RF-shield was modified to a cylindric steel connection piece between the chamber window and the housing of the scintillator, which results in less additional layers for the detected particles to interact. The entrance and exit window of the chamber for the neutron beam was still covered with 100µm aluminium foil for RF-tightness. In the experiment, sample data from C(n, lcp) and CH2(n, lcp) reactions were studied.
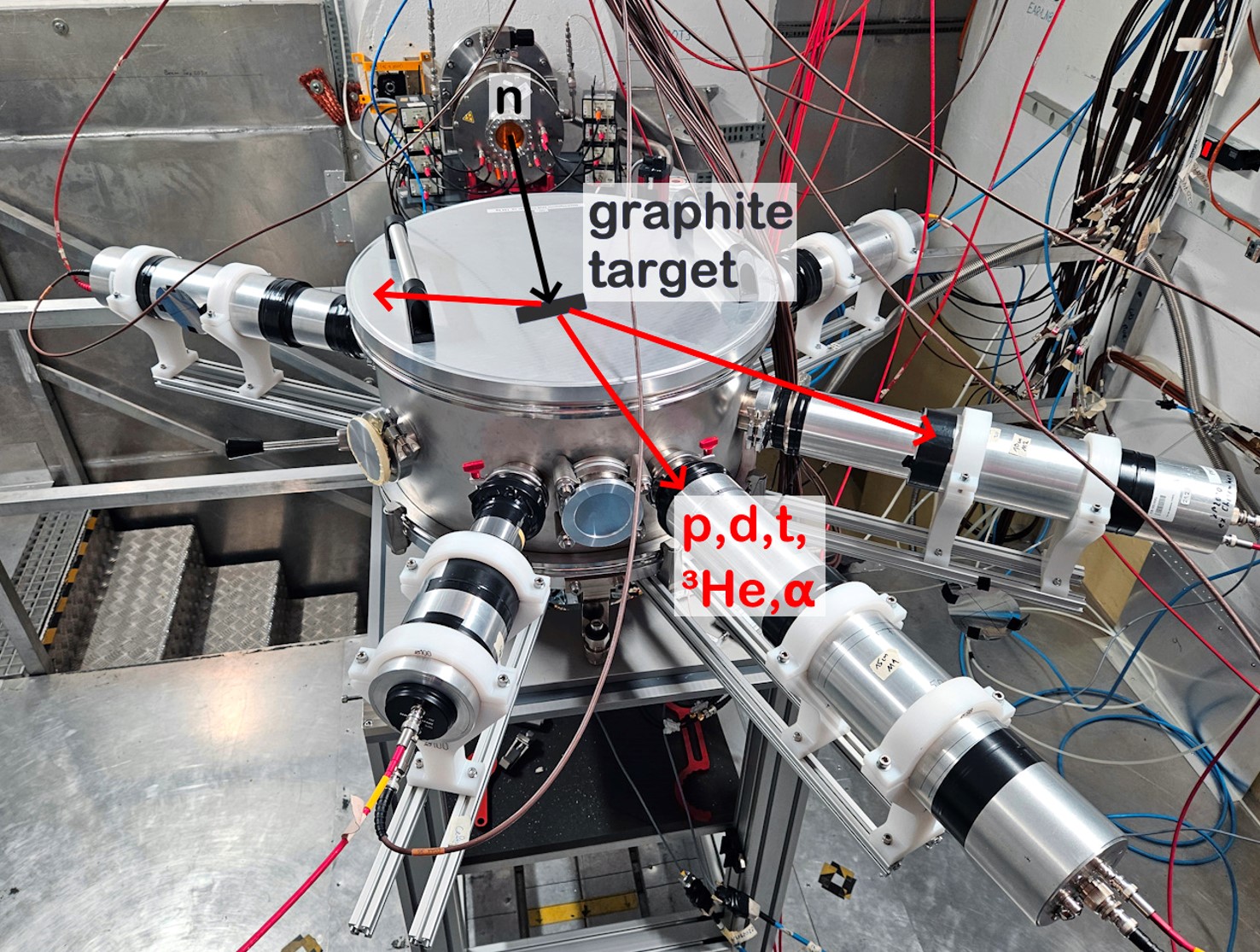
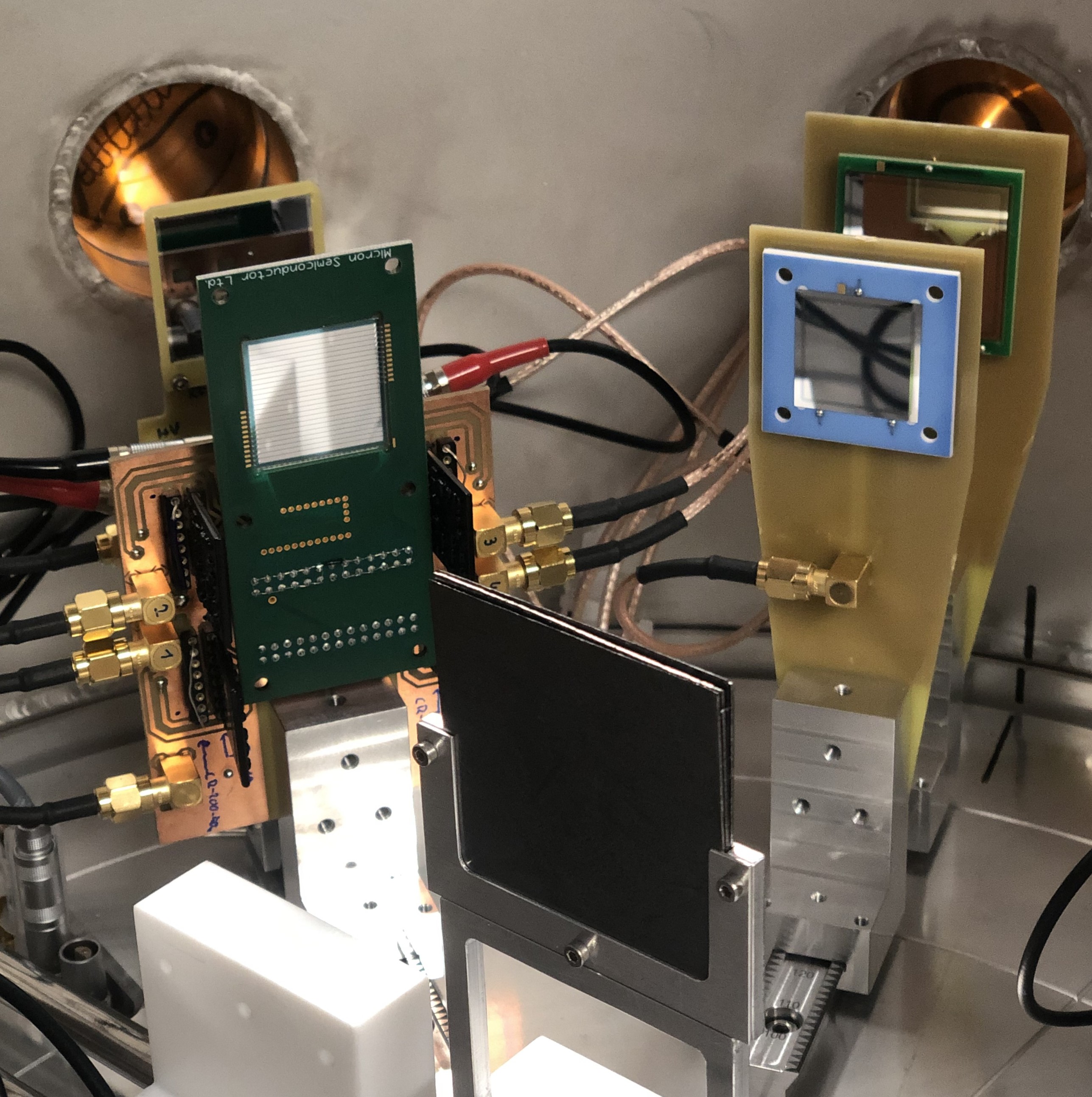
Figure 3: (Left) Experimental setup at n_TOF EAR1 with the new designed chamber and surrounding particle detectors. (Right) Example of silicon diodes and their holders as particle detector telescope inside new chamber with one segmented diode of 60µm on the left having four read-out channels with CR-110 modules. Their signals were further modified with Ortec 579 Fast-Filter Amplifiers.
Results
Figure 4 shows examples of wave form signals generated by 0.3 mm and 0.5 mm silicon diodes using CR-110 and CR-111 preamplifiers, respectively. The signals were further modified by Fast Filter Amplifier from Ortec 579 connected using 50 m long cables. Similar results were obtained by mounting together the CR-11x preamplifier with a CR200-50 shaping amplifier on the silicon diode board inside the scattering chamber. The absence of RF noise in the signal highlights how the new experimental setup, either by placing the preamplifiers inside the scattering chamber or by connecting the output signals to amplification modules located far from the experimental hall, proves to be optimal for shielding the signals from electromagnetic noise.
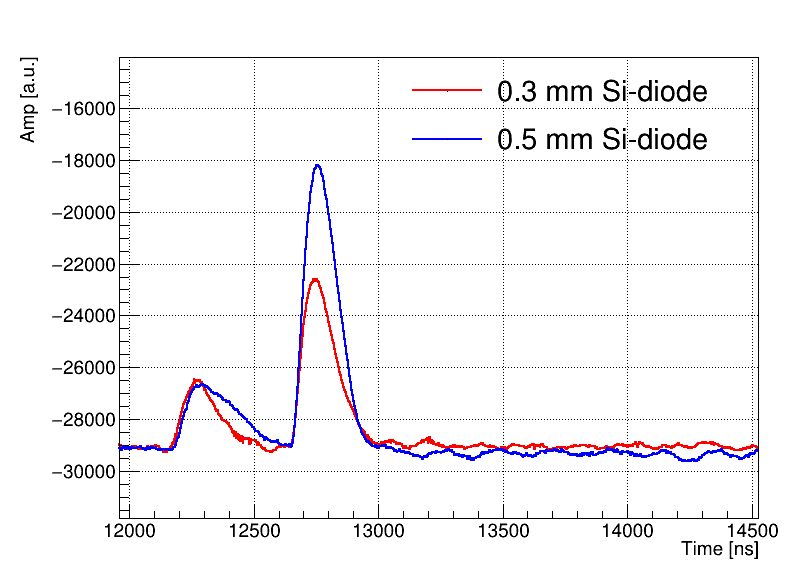
The signals generated by the silicon diodes are acquired and processed using a high-performance digital data acquisition system. Once recorded, the signals can be processed offline through the Pulse Shape Analysis routine [4] developed for the n_TOF experiments to extract information such as the time of flight of the emitted particles or the pulse height for each detected event. The signals produced by silicon detectors are selected if they exceed a defined minimum amplitude threshold. Subsequently, the time-of-flight information is used to search for coincidence events between pairs of detectors; if the difference between the times of flight falls within a predefined time window, the events can be considered as coincidences generated by the same particle passing through both detectors.
Figure 5 shows the distribution of the deposited energies by charged particles (labelled in the figure) in the silicon diode pairs S1-S2, consisting of 60 µm and 500 µm (top) and 300 µm and 500 µm (bottom), produced by the neutron interaction with the carbon target. An excellent separation of the different ionic species is observed, allowing for a clear and accurate identification of the detected particles.
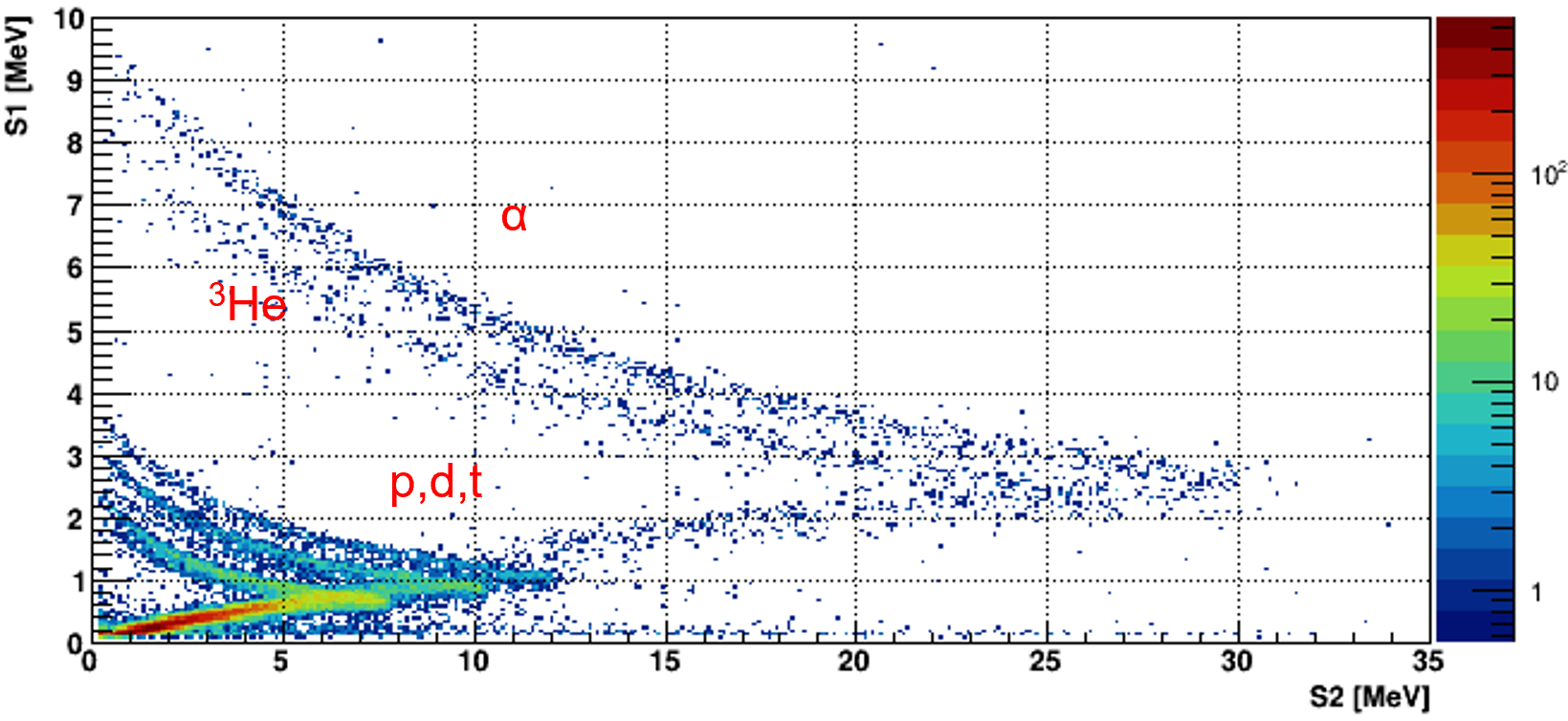
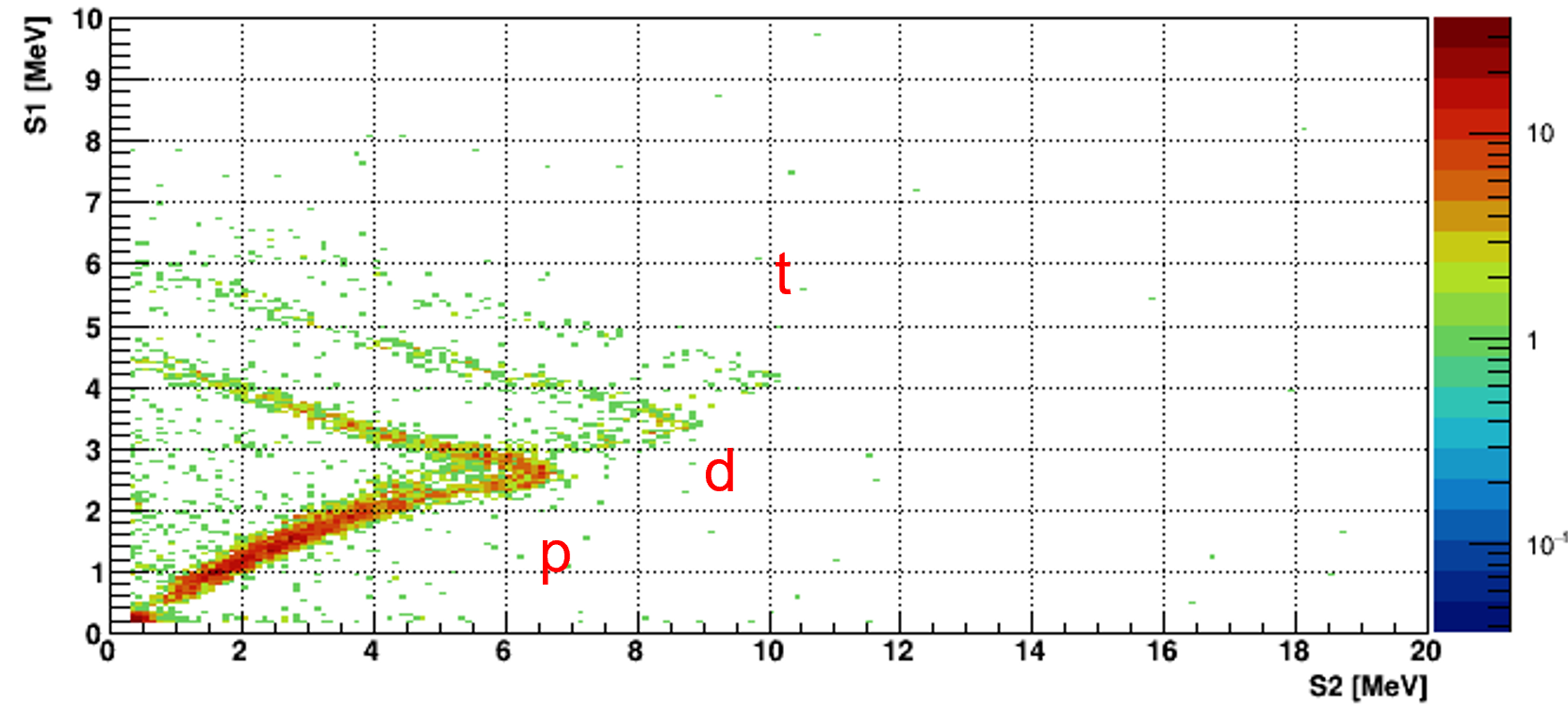
Summary
Employing semiconductor detectors at pulsed ultra-strong neutron sources, such as n_TOF, presents many challenges. Besides fast timing in the amplification, the pulse shape analysis was impeded by electromagnetic interferences induced by the gamma flash leading to RF-noise, in some cases higher than the signal from the particle-induced events of interest. The solutions vary greatly depending on the details of the experimental setup. In general, however, they mainly rely on the design of a RF-tight shield around the detectors and the electronics, in case inside a well-grounded vacuum chamber. In particular, the preamplifier modules and all cable connections need to be carefully shielded. With these findings, neutron-induced studies of (n, lcp) reaction are possible at pulsed ultra-strong neutron sources such as n_TOF (CERN) in the interesting neutron energy range above 100 MeV, as excellent identification of the different ionic species was achieved.
References
[1] R. Beyer et al., Letter of Intent INTC-I-221 to the ISOLDE and Neutron
Time-of-Flight Committee, CERN-INTC-2020-061, September 2020.
[2] M. Dietz et al., EPJ Web of Conferences 288, 01003 (2023).
[3] R. Beyer et al., Proposal INTC-P-651 to the ISOLDE and Neutron
Time-of-Flight Committee, CERN-INTC-2023-007, January 2023.
[4] P. Žugec et al., Pulse processing routines for neutron time-of-flight data. Nucl. Instrum. Methods Phys. Res. A, 812:134-144, 2016.