Laboratory web page: Horia Hulubei National Institute for R&D in Physics and Nuclear Engineering, IFIN-HH (external link)
The Target Preparation Laboratory from Nuclear Physics Department of IFIN-HH produces targets of various thicknesses made from both naturally occurring materials and stable enriched isotopes for nuclear structure experiments (nuclear structure and lifetimes measurements of excited nuclear states using γ-ray spectroscopy techniques: Doppler shift attenuation, plunger, and fast-timing, nuclear structure studies using the activation technique, cross-section measurements of interest for nuclear astrophysical processes, etc.) performed at IFIN-HH 9 MV Tandem Accelerator, at the 3 MV Tandetron and at international research facilities (CERN, IN2P3, TUM, IKP, JINR, etc). We have capabilities for target making and target characterization. For special cases we can easily work with laboratories that exist in other laboratories of IFIN-HH or of physics institutes from the same campus in Magurele. We also produce occasionally targets for external collaborators that do experiments at IFIN-HH or at outside facilities. Recently we entered a phase in which (i) we want to increase the number of procedures offered and (ii) we are preparing to produce targets for the specific needs of nuclear physics for astrophysics experiments: thick oxide targets for reactions on 16O, heat resistant targets for heavy irradiation, easy to characterize targets. For the latter we plan to cooperate with members of the WP3 of this ChETEC-INFRA project. Below we present the techniques and the equipment we currently use in the laboratory and some results.
Target Preparation Methodology
According with Table 1, during the last years a large variety of targets with specific features related to the experimental particularities, were prepared by using different methods, either available in the target preparation laboratory, or in collaboration with other laboratories, that have complementary equipment (e.g. PLD method) [1-5]
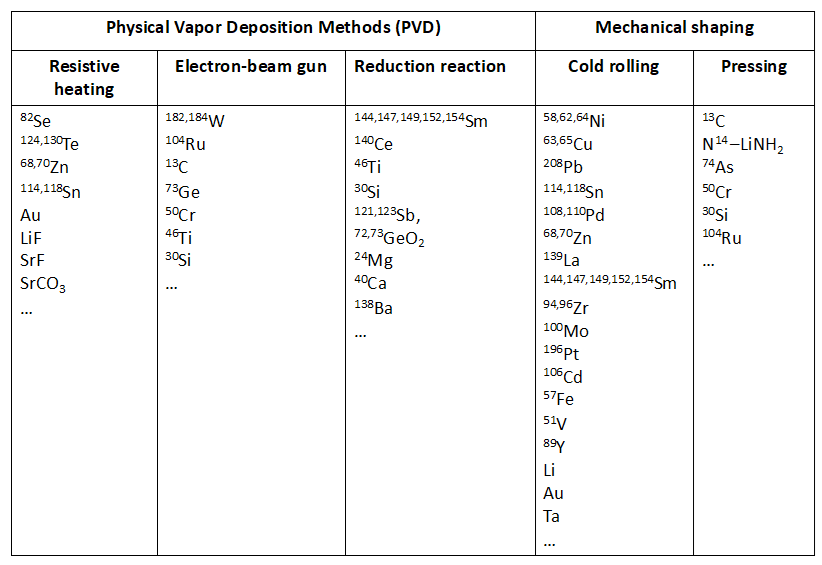
The choice of the method for target preparation depends on different parameters as the chemical or physical form of the starting material, the type of target (e.g. self-supporting or backed), or the target thickness. This makes it difficult to indicate, which preparation technique is the most suitable for a specific target. The method should assure the production of the final target with the required parameters, but as well should be efficient in terms of minimizing the costs, especially when made of very expensive enriched isotopic material. To optimize the consumption of the material before producing the final isotopic target the method is developed or tested with natural material.
To assure good target quality, the laboratory is endowed with high performance equipment that allow targets preparation by PVD – Physical Vapor Deposition methods (resistive heating and electron beam-based systems), cold rolling and tablet pressing techniques.
Using PVD methods the preparation of targets is carried in high vacuum. The material is evaporated from a heated suitable crucible and collected on a substrate placed above the vapor source. Through these methods, both self-supporting and backing supported targets with thicknesses in the range of up to a few hundreds of mg/cm2are prepared, but also deposits in the range of mg/cm2 are produced when needed. These methods allow production of layers with homogeneous material distribution over relatively large areas, however at the price of a very low collection efficiency and material loss.
The most commonly used PVD methods in the target preparation laboratory of IFIN-HH are resistive and electron beam heating.
Using the resistive heating method, materials with melting point lower than 1800°C can be evaporated from boat- or tube-shaped crucibles placed between water-cooled electrodes. The used crucible’s melting point has to be much higher than that of the evaporated material, otherwise a high amount of impurities will be introduced into the target deposit. Boats made of the refractive metals Ta, Mo, W are mainly used.
For materials with higher melting points, electron beam heating is used. In the electron beam heating method, the evaporated material is placed in a water-cooled crucible (made from Ta, Mo, W, C, BN) and is heated by a focused beam of electrons emitted from a tungsten filament.
The laboratory includes also equipment for target preparation by cold rolling and tablet pressing methods.
Cold rolling is considered the primary methods of target preparation, especially for expensive enriched isotopes as the waste of material with this technique is minimal and the method is known for yielding a high-quality target. It is extremely efficient for self-supported metallic foil production using small amounts of starting material. In this method the material is transformed into foil using a rolling mill. To protect the material from collecting impurities from the rollers, the material to be rolled is placed in a rolling pack made of stainless-steel sheet. The achievable thickness of the final product varies a lot from material to material depending on its malleability. In general, the lower thickness limit for most of the metals is at the level of ~ 1 mg/cm2. It is much more difficult to achieve a low thickness with soft materials. The practical limit is around 2 mg/cm2 but in some cases even 2.5 mg/cm2 is achievable only with considerable effort.
Tablet pressing method is in general used for self-supported thick targets preparation. In case of very hard materials that can’t be pressed into a tablet, the addition of a binder can be a solution, if acceptable by the experiment. For the preparation of thick targets for medical radioisotopes production, which are able to withstand the bombardment by an intensive beam, hot pressing or sintering procedures can be applied.
Equipment for Target Preparation
Two PVD systems with resistive heating and electron beam-based systems are available, and shown in Fig. 1.
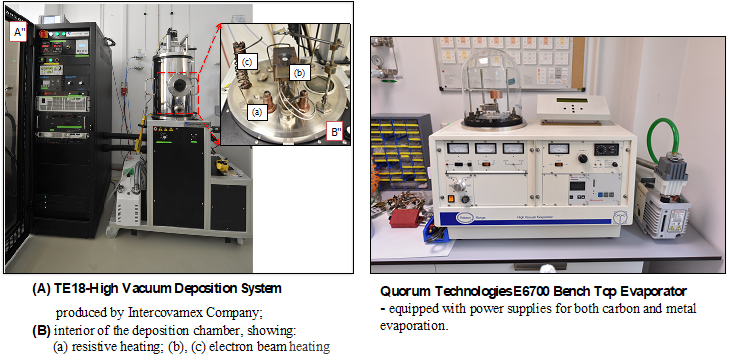
(Credit: IFIN-HH)
2 electrically controlled Durston type rolling mills with variable speed and equipped with rolls made of specially selected roll steel, heat-treated on the main working surface.
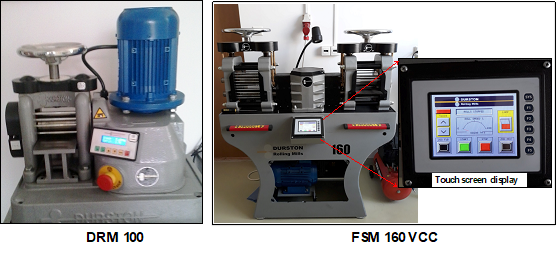
(Credit: IFIN-HH)
Specac automated hydraulic presses (25T) – equipped with 40 mm, 32 mm, 20 mm, 13 mm, 20 mm and 5 mm evacuable pellet dies, manufactured from hardened stainless steel for optimum quality and durability, and highly polished surfaces for contact with the sample.
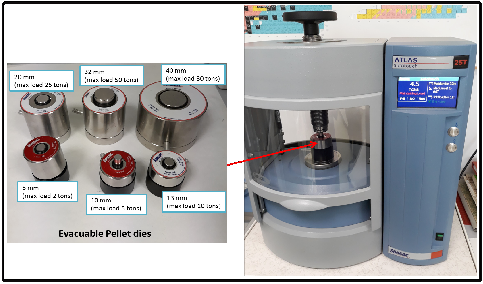
(Credit: IFIN-HH)
Other auxiliary equipment is also used to provide support for the numerous other activities required for target fabrication such as:
- Mettler Toledo XP56/M analytical balance with 6 decimals digits and a weighing capacity of 52 g with 0.001 mg readability;
- Nabertherm tube furnance equipped with a gas supply system for protective gas or vacuum operation which can be operated at temperatures up to 1300 °C;
- Ohaus Micro Centrifuges for high-speed laboratory applications as ultrafiltration;
- Ohaus Incubating Orbital Thermal Shaker for heating applications that require consistent and precise high-speed shaking;
- Labconco inert gas glove box for handling of targets that may oxidize quickly;
- Oven, vacuum oven, constant climate chamber (Memmert type), etc.
For the preparation of high-quality targets with selected properties that perfectly answer nuclear physics experiments requirements, different approaches and experimental protocols are currently applied:
(a) Self-supported 13C, 14N, 30Si, 16O were prepared by tablet pressing method using the Specac automated hydraulic 25T press (Fig. 4).
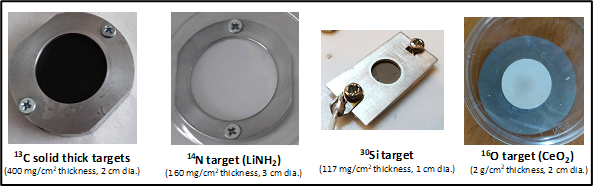
(Credit: IFIN-HH)
(b) Another method involves metallothermic reduction reaction of different oxides with dedicated reducing agents (Zr, Hf, La, CaH2, Mg, C, H2), which subsequently leads to obtaining of high purity metallic form of the deposited thin film (144,147,149,152,154Sm2O3, 140CeO2, 46TiO2, 30SiO2, 121Sb2O5, 72,73GeO2, etc.). Reliable thin films made of natCa, natBa, natSr, 24Mg in metal form that can resists oxidation by air are also produced (Figure 5).
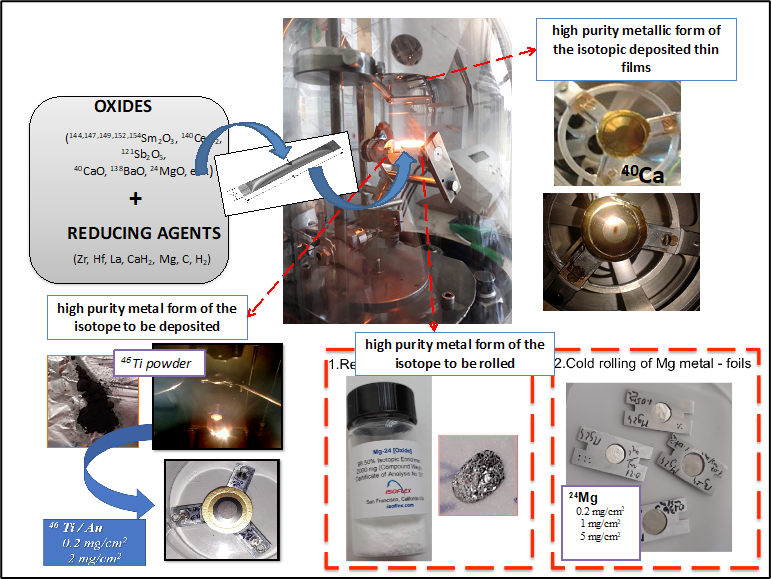
(Credit: IFIN-HH)
c) To produce reliable thick targets from refractory materials as Os, W with extremely high vaporization temperatures (4500-5500°C) using Pulsed Lased Deposition (PLD) technique was also developed in collaboration with the National Institute for Laser, Plasma and Radiation Physics (INFLPR) [3].
(d) Also, in the same laboratory, to remove the oxygen layer (as contamination) from the surface of different metallic isotopic foils (64Ni, 65Cu, 208Pb) resulted during the fabrication process a thermal treatment using a hydrogen oven was successfully applied. The process of removing the surface oxygen contamination is very useful for sub-barrier neutron transfer reactions with 18O or 13C beams [4].
(e) Other self-supported or backed targets were also produced through high vacuum evaporation methods or by mechanical rolling: 130Te, 182,184W, Au, Ta, Y, Nb, Sn, SrF2, etc (Figure 6) [5].
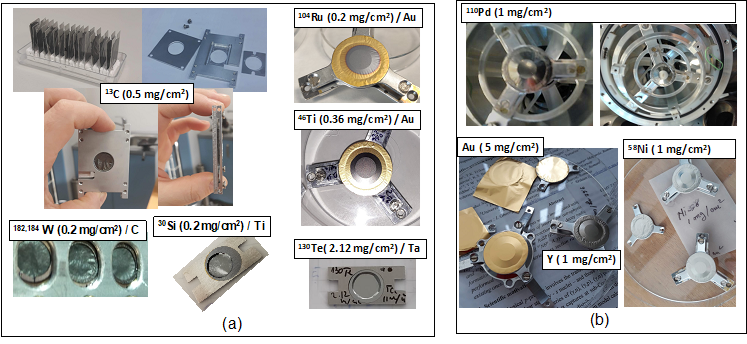
(Credit: IFIN-HH)
Target Characterization Techniques
The target characteristics, quality and reliability are important for the experiments’ feasibility in the first place, but also for the degree of confidence in the assumed accuracy. In this context, the obtained targets are characterized in terms of thickness, phase composition, crystalline structure, chemical purity and morphology by using multiple advanced techniques such as RBS (Rutherford Backscattering Spectrometry), PIXE (Proton Induced X-ray Emission), PIGE (Proton Induced Gamma-ray Emission), XRD (X-ray Diffraction), OM (Optical microscopy), AFM (Atomic Force Microscopy) and SEM-EDX (Scanning Electron Microscopy and Energy Dispersive X-ray Spectroscopy).
Target characterization @ 3 MV Tandetron™
Nuclear physics research ongoing in our institute for more than four decades was strengthened in 2010 when a 3 MV Tandetron™ accelerator system has been installed and commissioned at IFIN-HH [6]. The accelerator system was developed by High Voltage Engineering Europa B.V. (HVE) and comprises three high energy beam lines (Figure 7). The first beam line is dedicated to ion beam analysis (IBA) techniques: RBS, Nuclear Reaction Analysis – NRA, PIXE and PIGE and micro-beam experiments – μ-PIXE. The second beam line is dedicated to high energy ion implantation experiments and the third beam line was designed mainly for nuclear cross-sections measurements used in nuclear astrophysics. A unique feature, the first time in operation at an accelerator facility is the Na charge exchange canal (CEC), which is used to obtain high intensity beams of 4He− of at least 3 μA. The potential of this new facility was achieved in various fields, from IBA to radiation hardness studies and from medical or environmental applications to astrophysics [7]. The 3 MV Tandetron™ can deliver beams of protons and 4He, for RBS, with electrical beam currents ranging from 1 nA up to 100 nA on target.
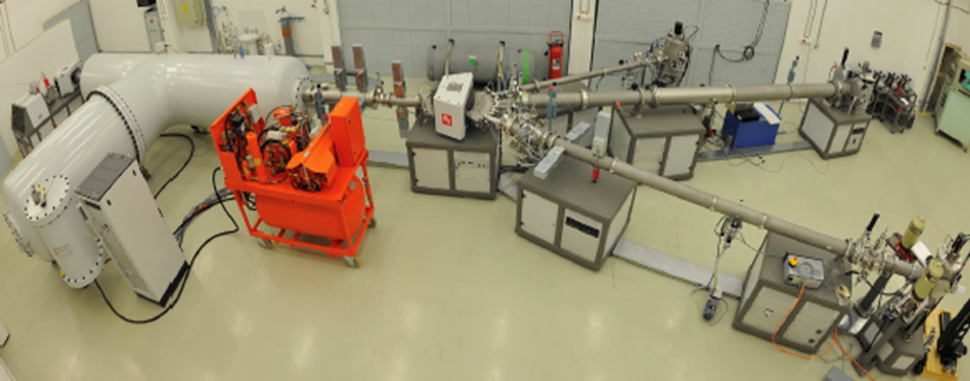
(Credit: IFIN-HH)
It appears the manufacturing process combined with the characterization techniques offer a strong perspective for target development methods.
Nuclear Astrophysics @ IFIN-HH – Experiments @ 3 MV Tandetron: study of ion-ion fusion mechanisms at very low energies
The Nuclear Astrophysics Group (NAG) at IFIN-HH has been performing tests to study the reaction 12C + 16O, as part of a program focused on ion-ion fusion reactions at very low energies. The aim is to use a neighboring reaction, such as 13C + 16O or 12C + 17O, and rely on the activation method to determine partial cross-sections, and therefore the total fusion cross-section. We produced for them thin 13C targets, but it turned out that they do not resist for intense beams. Next, we produced 16O targets as Ce-oxide (CeO2). They resisted in-beam, and the experiments were successful. We also made and tested targets containing F: LiF and BaF2 targets and their suitability was tested.
1) Measurement of the Activation Channels Produced with the Reaction 13C+16O
A. Carbon-13 targets
The first test done was with an 16O beam on a 13C target. The 16O beam was produced easily at lab energies between 10 MeV and 14.8 MeV with currents between 3 pμA and 240 pnA, respectively. Two 13C targets were prepared by deposition of 13C on Ta backing (Figure 8).
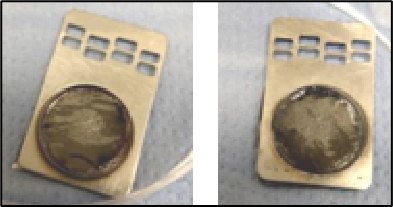
(Credit: IFIN-HH)
The thicknesses of the deposited 13C layers were determined by using RBS method, as ~130 nm and ~150 nm. Given the short half-lives of the nuclei produced (27Mg with T1/2=9.8 min, 28Al with T1/2=2.2 min), the targets were used for repeated measurements of 30 – 60 mins irradiation followed by 50 minutes decay measurements. The targets held well for irradiations with currents less than 1.5 pμA, however it was found that at higher currents they started exhibiting a silver-tinted beam spot (see Figure 9).
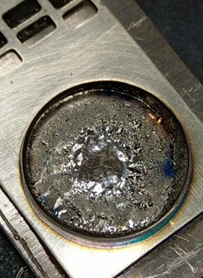
(Credit: IFIN-HH)
Initially, we suspected that this was only due to heating effect created by the beam impact. However, the flange holding the target was kept cool with a water-based system during irradiation. A more detailed look at the surface with SEM revealed that the silver-tinted spot was in fact the Ta backing and that the 13C layer in that spot was gone (Figure 10).
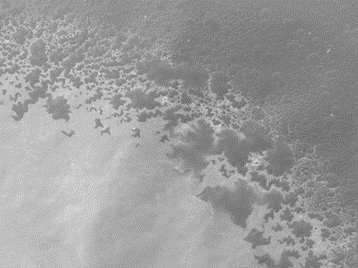
(Credit: IFIN-HH)
Given these test results and that the NAG group’s interest is in measurements at very low energies, and low cross-sections, where high beam currents are paramount it was decided that such thin targets could not be used to measure the reaction of interest.
B. Oxygen targets
The second test done was with an 13C beam on a 16O target. A beam of 13C was used at different lab energies between 8 and 15 MeV. The currents produced also varied between 3 pμA and 200 pnA, respectively.
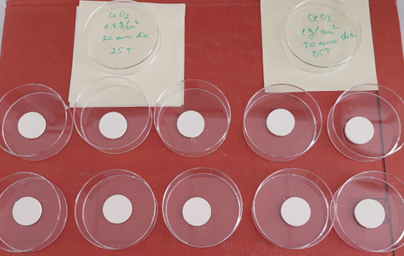
(Credit: IFIN-HH)
The necessary oxygen targets were created from cerium (IV) oxide powder, CeO2. This type of oxide was chosen because cerium has a high number of protons and as such a high Coulomb barrier, therefore will have a very low probability of interaction with the beam. The powder was pressed into pellets under 25 T pressure. The diameter of the pellets was fixed at 2 cm or less in order to fit inside the target holder, but different thicknesses were attempted: 0.8 mg/cm2 and 1 mg/cm2. It was found that the targets could not be made any thinner than 0.75 mg/cm2 as they became too fragile to handle and crumbled immediately. Figure 11 shows a set of 10 targets with two of the mentioned thicknesses. In order to provide support and easier handling, the pellets were attached to aluminum backing in the shape of discs (as can be seen in Figure 12).
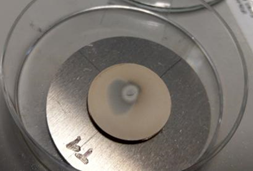
(Credit: IFIN-HH)
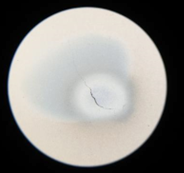
The targets were irradiated on average for 60 minutes and were affected on various levels by the beam depending on the current. Figures 12 and 13, show target T1 (0.8 mg/cm2) after 40 min of irradiation with 13C at 10.8 MeV and a current of ~80 pnA.
Similarly, Figure 14 shows T8 (0.8 mg/cm2) after a first irradiation of 60 minutes with a beam current of ~1pμA. It can be seen that the beam spot area is affected much stronger due to the higher intensity. It should also be noted that in this case, it was not possible to start the irradiation at maximum beam intensity because the impact with the target would cause the vacuum to break. Instead, the initial current was ~100 pnA and it was then increased slowly to allow for the operational vacuum pressure of 10-6 mbar to be maintained.
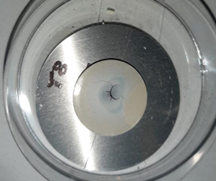
(Credit: IFIN-HH)
This was considered a better option than the previously described 13C targets and as such were used for a 5-day long experiment to measure the yields and cross-sections of the 13C + 16O reaction for ELAB = 8-15 MeV, in energy steps of 0.4 MeV.
Over the length of the experiment, it was determined that the pellets could be irradiated a maximum of 3 times at the higher currents before they became unusable and up to 5 times at intensities on the order of ~250 pnA. Figure 15 shows target T4 as an example of the first case, where 3 irradiations were done at high intensities.
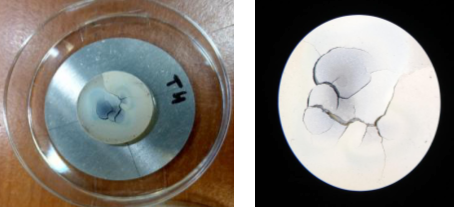
(Credit: IFIN-HH)
We suspect that the damage sustained by the pellets was due to the heat produced because of the high beam intensity, even though the target holder was equipped with a water-cooling system. We have also found that if the targets are held in vacuum for at least a day before irradiation, they perform better. Finally, it appears that the best option for such targets is for them to be used for a single measurement/irradiation, where the material availability permits this.
2) Measurement of the Activation Channels Produced with the Reaction 13C+19F
As part of their ion-ion fusion reactions program, the NAG group also intends to study the reaction 12,13C + 19F at very low energies. Given that the option of 13C targets has already been tested, as shown in the previous section, the focus for this reaction was on testing two types of fluoride materials: LiF and BaF2. Unfortunately, due to time constraints on the beam availability it was not possible to perform extensive tests.
A. LiF targets
The first test was done with LiF targets. They were made from LiF powder pressed into pellets at 10 T pressure. The diameter of the pellets was 1.3 cm and they were also placed on aluminum circular disks for easier handling (Figure 16).
One issue that was immediately apparent was that they outgassed more strongly than their CeO2 counterparts and as such it took longer to reach the intended beam current during irradiation, even when for relatively low intensities. For example, Figures 16 and 17 show a LiF target after 1.5 hrs of irradiation with ~50 pnA. The beam spot is highly visible but the target held well.
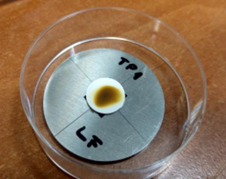
(Credit: IFIN-HH)
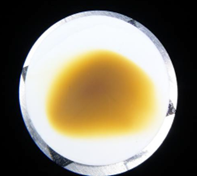
(Credit: IFIN-HH)
However, for the purpose of the 13C+19F reaction measured, it was decided that the Li component created too much contamination in the prompt spectrum and it both overshadowed the channels of interest as well as endangered the gamma detectors.
B. BaF2 targets
The second test was done with BaF2 targets. They were made from BaF2 powder, also pressed into pellets at 10 T pressure. Barium has a much higher proton number and as such the issue of contamination in the spectrum was eliminated. The diameter of the pellets was also 1.3 cm and they were placed on rectangular aluminum backings for easier handling (Figure 18).
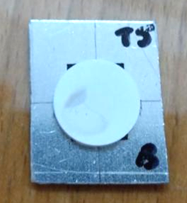
The issue here was that they appear to be more fragile than the LiF ones. Two targets broke just from handling, one before irradiation and one after irradiation (during transport to the decay measurement station). It’s possible that a larger diameter for the pellets (which will allow a maximum pressure of 25T) will make them sturdier and it’s an option to be tested in the future. Further testing will also be required to determine how these pellets hold at higher currents and longer irradiation times.
References
[1] N.M. Florea, L. Stroe, R. Marginean, D.G. Ghita, D. Bucurescu, M. Badea, C. Costache, R. Lica, N. Marginean, C. Mihai, V. Mosu, C.R. Nita, S. Pascu, T. Sava, J Radioanal Nucl Chem 305, 707 (2015).
[2] A. Mitu, N.M. Florea, N. Marginean, R. Marginean, Gh. Cata-Danil, Manufacturing and characterization of targets at IFIN-HH: developing an interdisciplinary body of knowledge, EPJ Web of Conferences, 229, 03001 (2020)
[3] A. Mitu, M. Dumitru, R. Suvaila, A. Oprea, I. Gheorghe, P. Mereuta, S. Brajnicov, I. Burducea, N.M. Florea, N. Marginean, T. Glodariu, M. Dinescu, G. Cata-Danil, Vacuum 161, 162 (2019)
[4] A. Mitu, M. Dumitru, F. Dumitrache, N. Marginean, R. Suvaila, C. Nita, M. Dinescu, Gh. Cata-Danil, High grade decontamination of Ni targets for sub-barrier transfer reactions, U.P.B. Sci. Bull., Series A: Applied Mathematica and Physics, 80, 199 (2018)
[5] A. Mitu, A. Oprea, M. Dumitru, N.M. Florea, T. Glodariu, R. Suvaila, C. Luculescu, N. Marginean, M. Dinescu, Gh. Cata-Danil, J Radioanal Nucl Chem 316, 725 (2018).
[6] I. Burducea, M. Straticiuc, D.G. Ghiță, D.V. Moșu, C.I. Călinescu, N.C. Podaru, D.J.W. Mous, I. Ursu, N.V. Zamfir, Nucl. Instrum. Methods Phys. Res. Sect. B Beam Interact. Mater. Atoms 359, 12 (2015). https://doi.org/10.1016/j.nimb.2015.07.011
[7] G. Velişa, R. F. Andrei, I. Burducea, A. Enciu, D. Iancu, D. A. Mirea, A. Spiridon, M. Straticiuc, Eur. Phys. J. Plus 136, 1171 (2021). https://doi.org/10.1140/epjp/s13360-021-02156-7